Deep in the bowels of a large brick building on the WSU campus is a laboratory guarded by red flashing lights and warning signs. A tiny window in the door offers glimpses of stainless steel machinery while a low pulsating hum emanates through thick concrete walls.
Inside the W. M. Keck Antimatter Laboratory, a deuteron accelerator produces up to 120 billion positrons per second—about 10 trillion positrons per day, more than any other university or small laboratory in the nation.
Positrons are a type of antimatter. For every proton, neutron, or electron spinning within an atom there is a particle of opposite charge—its antiparticle. Positrons are the antiparticle of electrons. When the two collide, they annihilate releasing one hundred percent pure energy in the form of gamma rays.
It’s a type of energy that could one day propel starships far beyond the solar system.
“We can create antimatter very easily in the matter world,” says Kelvin Lynn, director of the Keck laboratory and Regents Professor in physics and mechanical and materials engineering, “It just doesn’t live very long because it eventually finds an electron and annihilates. But if you put it in a vacuum, it will live forever.”
Confining positrons safely within a vacuum, however, is a monumental problem, as is harnessing energy from their annihilation. But for Lynn, impossibilities are the stuff of life.
A pioneer in the world of antimatter since 1975, Lynn was one of the first to use a magnetically-guided positron beam. In 1996, he accepted the Boeing Chair of Advanced Materials at WSU and promptly traded New York for Pullman.
Marc Weber, research professor and Keck lab manager, joined him in 1997. As a graduate student, Weber had worked on antimatter with Lynn at Brookhaven National Laboratory. He then returned home to his native Germany. Once Lynn arrived at WSU, he invited Weber back to rebuild their research program.
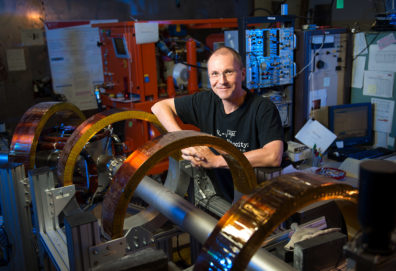
(Photo Robert Hubner)
Together, they brought in Lynn’s old equipment from Brookhaven and constructed WSU’s first positron beam in the physics department. After four years of extremely difficult work, they were rewarded with a $1.1 million grant from the prestigious W.M. Keck Foundation to build the accelerator that would set a new standard for antimatter research.
In no time they were achieving milestones never before possible.
Their innovative research soon caught the eye of the U.S. Air Force. In 2006, the military challenged Lynn to find a way to trap and transport positrons, specifically by storing them in plasma—a unique type of ionized gas. Safely storing antimatter would be the first step toward using it for propulsion and ultimately, deep space travel.
Lynn accepted the dare with his trademark intensity. But like others before him, he couldn’t overcome the repulsive forces that occur when a billion or so positrons are squeezed together into a plasma container, or “trap.”
Particles of like charge repel each other, so an enormous amount of energy was required to hold the positrons together in a small space. They estimated about a million volts of electricity, a prospect much too expensive and not at all portable.
Stymied, Lynn reluctantly joined the ranks of scientists who were foiled by the repulsion problem. In the world of physics, the general consensus was that it was impossible.
Deeply disappointed, he couldn’t stop thinking about it and one restless night, drifting in and out of sleep, Lynn had an epiphany. Rather than trying to compress billions of positrons into a box-like container, they could instead give them “breathing room”—line them up side-by-side in an infinitely long and narrow vacuum tube.
From there, he realized, the tube could be cut up into tiny straws—each containing just one positron. The concept was unprecedented and offered the world its first practical means for storing and transporting antimatter.
The Air Force was elated and the project was funded. Lynn’s team went on to design a prototype trap, about the size and shape of a soda can.
“I’m embarrassed it took me so long to figure it out,” Lynn says. “It’s so simple.”
Today, employees in the antimatter lab keep to an intense schedule, hidden here and there within a maze of towering monitors, banks of electronics, tool benches, and radiation detectors.
Each one works on a specific piece of the trap such as creating the perforated disks that Weber calls “holey wafers.” Two hundred of these small disks must be perfectly aligned to form the “holey tubes” where the positrons will be stored. Using special mirror-like coating, magnetic fields, and electric barriers, up to two billion positrons are expected to live peaceably side-by-side in the 20,000 tubes within the soda can trap.
Weber concedes the work is extremely challenging. “It pushes the limits of everything Kelvin knows,” he says. “It pushes the limits of what I can do by far.”
The process of studying antimatter begins with creating the antiparticles themselves. Each miniscule positron comes to life in the accelerator when three million volts of electricity smash deuterium ions into a piece of graphite, producing an isotope which decays into antimatter.
Like distracted toddlers, these newborn positrons tend to scatter in all directions. Lynn compensates with a special type of funnel that corrals the particles into a narrow beam.
The beam is spiraled through a long vacuum tube, guided by a magnetic field, until it is ushered into the tiny tubes in the trap. The trap itself is housed within a super-cooled, superconducting magnet, the size of an MRI machine.
“Right now, we are working with electrons,” says Weber. “We let them go through the system and store them; then let them out the back door, and count how many are left. You can see them individually on our computer screen.”
So far, they have stored electrons for up to one second. The goal is to store them in the trap for hours, even a day. Once they are successful with electrons, they will try it with positrons.
“For starship travel, we want to store positrons for hundreds of years,” Weber says. “In principle, it should be possible.”
If successful, their work would open an entire new field of possibilities, including large-scale production of antimatter fuel. For example, two tiny sand grains of antimatter could provide the same amount of energy as 1,700 tons of liquid hydrogen-oxygen fuel once used to power the Space Shuttle. Only two 9-volt batteries are needed to power the trap itself.
Lynn speculates that, “with a few grams of antimatter and a ten-pound trap, astronauts would indeed be able to fly beyond the solar system.”
“You would open the door in the trap and carefully allow minute amounts of antimatter to annihilate. The resulting gamma rays would push a starship faster and faster until it was nearing the speed of light,” he says.
Dreams of flying into the wilds of space are as ancient and primal as the earliest stargazer and Lynn and Weber have laid the groundwork that could eventually make it so. No one beyond the realm of science fiction has come as close to harnessing antimatter’s phenomenal power.
Weber says the day is imminent. Gradually taking the reins from Lynn, Weber will soon carry the research forward as fulltime program director. If all goes well, he says his graduate students could see the promise of antimatter energy fulfilled within their lifetimes.
Read about Kelvin Lynn’s life and his work with making the crystals necessary for antimatter research, in “Paths that grew crystal clear.”