Lai-Sheng Wang has an impish smile, an infectious laugh, and a high-powered research program that studies matter a few atoms at a time.
He uses massive machines to create tiny clusters of atoms. Wang’s clusters aren’t mere lumps. As the magnetic models stuck to his file cabinets show, they are as geometrically elegant as a snowflake.
Wang came to the public’s attention three years ago when his team at the Pacific Northwest National Laboratory in Richland became the first to make golden buckyball, a hollow cage of 16 to 18 gold atoms. He has also worked on clusters of aluminum, another element with a long track record of useful applications.
Now Wang is devoting himself to the study of boron.
Boron? Really?
Boron is not what you’d call a high-profile element. It is rare on earth, but not rare enough to be especially valuable. Plants need it to build strong cell walls. Humans might need it, but in such small amounts that we get enough just by eating and breathing. It has a few industrial applications.
On the surface, boron doesn’t appear to be all that interesting.
So why study it?
Because it comes from an interesting neighborhood. On the periodic table of the elements, it’s right next door to carbon. That means it’s almost the same size as carbon and shares many of the same properties. Carbon, of course, is plenty important; it’s the basis of life on earth. (Aluminum is also nearby, as is silicon, so important to the electronics industry. This is a very important neighborhood.)
In nature, boron is always bound to some other element, usually oxygen. Wang wants to find out what structures boron alone can form and what their properties are. Different structures will behave differently, just as the different forms of carbon do. Both diamond and graphite (the “lead” inside a pencil) are made entirely of carbon atoms. In diamond, the hardest naturally-occurring material on earth, they are in a rigid crystal arrangement. In graphite the carbon atoms are arranged in a hexagonal pattern, like chicken wire, with many flat layers of the hexagons piled up in a stack.
“That makes graphite so unique,” says Wang. “It’s a very hard material in one direction, but very slippery in the other direction. That’s why you can write with your pencil, scratching off the graphite in these layers.”
In other words, form is crucial; and form is what Wang creates and studies. His lab is crowded with metal chambers and tubes, lasers, bundled wires, and throbbing machines. To make boron clusters, a small sample of natural boron is placed in a vacuum chamber and bombarded with a laser beam, which jars groups of boron atoms free from the sample’s surface. A jet of helium carries the fragments down a 12-foot-long vacuum tube with a chamber at the end, where another laser knocks an electron out of each cluster. A spectrophotometer then measures the speed of the departing electron, which indicates the energy state of the electrons still in the cluster. The distribution of electron energies, called a photoelectron spectrum, is the raw data that Wang and his colleagues use to figure out how the atoms in the cluster are arranged. It’s not a simple process; the researchers need sophisticated mathematics and a super-computer to analyze the peaks on the spectrum and tease out the structure that gave rise to them.
Wang often has some idea what to expect because he works closely with theoretical physicists whose mathematical calculations suggest what the most stable form of a certain-sized cluster will be.
A given number of atoms in one cluster can combine in many different ways. Usually, only one form will be energetically stable enough to survive in the lab. The others are so unstable they degrade almost as soon as they form. “Experimentally you just don’t see them. At the end [of the experiment], you just see the most stable one,” says Wang.
Sometimes the theoretical prediction fails. The most stable form of boron-9 was predicted to be a ring of eight boron atoms radiating out from a ninth in the middle. Wang calls the structure a “molecular wheel.” When his group analyzed the electron spectrum of boron-9, they found peaks that corresponded to a molecular wheel—and several that didn’t. Another PNNL scientist and a student re-did the math, and discovered that the method of calculation used for the initial prediction was wrong. It had not recognized that alongside the wheel-shaped cluster, and almost as stable, would be an oval structure with two central atoms surrounded by a ring of seven.
“This is why they are not going to displace experimentalists any time soon!” Wang says, laughing. “You do need the experimental data to validate the calculation.”
His group has worked its way through boron clusters containing up to 20 atoms. Now they’re beginning to explore larger clusters.
“Our progress has been slow because we are dealing with bigger and bigger systems, and the calculation becomes more and more difficult,” he says. In large clusters the electrons get crowded, their spectra are harder to decipher, and the number of possible arrangements of the atoms increases exponentially.
The effort is worthwhile both for the basic knowledge of quantum chemistry it will provide and for the potential payoff: Because of their chemistry, boron structures could be good at safely storing hydrogen fuel.
So far, none of Wang’s boron clusters can survive outside of the vacuum tube. He hopes one of the larger clusters will turn out to be as sturdy and stable as buckyball, the soccer ball-like cluster of 60 carbon atoms that was discovered in the 1980s.
Buckyball looks cool. It has also sparked a technological revolution. When they’re opened up into flat sheets, buckyballs form graphene, which looks much like naturally-occurring graphite. Roll a single layer of graphene into a tube and you get a nanotube, the microscopic structure that has become a pillar of the nanotechnology industry.
“Our research is very much in that spirit,” says Wang. “We’d like to discover something which can survive in the real world, like the buckyball. Buckyball was discovered in exactly the same way, but it turned out that it is super stable. You can put it in a bottle.
“I don’t think we have discovered such a thing yet. But that remains one of our dreams.”
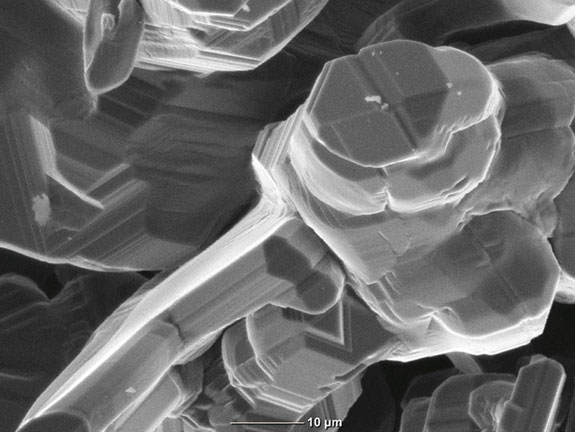