It’s been decades since I was asked, “What do you want to be when you grow up?,” but now I’m facing a mid-life version of the question, this one tinged more with fear than with hope. I just turned 53 and my current answer to the question of what I want to be in 20 or 30 years boils down to three things: healthy, solvent, and of sound mind.
Of the three, the one I worry about most is the last. I’ll have support networks to help me work around the others, if need be, but if my mind goes–
The fear, of course, is of the big A: Alzheimer’s disease. The most common cause of dementia, Alzheimer’s already afflicts more than four million Americans. By 2050, with the aging of my fellow boomers, that number is expected to triple. And there’s not much we can do about it. A few drugs are available that help in the early stages of the disease; but for the foreseeable future, to come down with Alzheimer’s is to step into an abyss.
Several scientists at Washington State University are exploring how memory works and what’s gone wrong when it doesn’t. I set out to talk with them about their research and, I hope, pick up tips about what I can do now to improve the odds that when I’m 80 my brain will still be healthy enough to let me cook my own meals, pay my own bills, enjoy my friends and family, and understand what’s going on in the world.
My first visit is with psychologist Maureen Schmitter-Edgecombe, who explores the borderland between normal functioning and the beginnings of dementia. Doctors call it MCI, for Mild Cognitive Impairment. She says MCI is not the same thing as early-stage Alzheimer’s, although the term is sometimes used that way. It’s just a label assigned to people who do poorly on certain tests of memory and routine thinking.
“Some would argue that people in this category really are early stage dementia,” she says, “but depending on how you get your sample, some of the people that fall into this stage actually revert back to looking like normal older adults the next time you test them a couple of years later.”
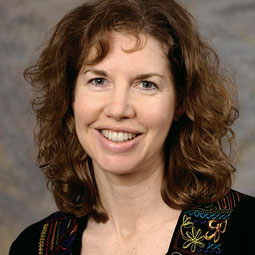
In her lab, simply failing a word-recall test doesn’t land you in the MCI category. Schmitter-Edgecombe is working to develop better ways to assess memory problems. Are a client’s memory lapses normal age-related glitches, or signs of an irrevocable slide into neverland?
“[People] can’t get in that category just because they had one poor test,” she says. “They have to be reporting a history of difficulties, the significant other has to be reporting a history of difficulties, and then all that information has to make sense with what we’re seeing in the testing data.”
In one test, she asks each client to do eight memory-related tasks over the course of an hour. The client is also asked to remember that after each task, he should remind a third person to take medication. At the end of the hour, Schmitter-Edgecombe asks the client to describe the eight tasks (not in detail, just well enough for her to recognize each one)–and to tell what order they came in.
I can imagine getting the first one and maybe the last two in the right order (assuming I could even recall all eight), but the ones in the middle? That sounds hard.
She says it’s not hard at all for young adults, “but as we get older, we do start to see changes.” Recalling the order in which events happened, or temporal order memory, is distinct from recalling the events themselves, which is called content memory. It’s possible to remember an event clearly but forget when it occurred, says Schmitter-Edgecombe. So far, most tests for Alzheimer’s focus on content memory: You’re asked to recall a list of words, who’s President, or what you ate for breakfast. But such tests miss non-content aspects of memory that may be even more important to everyday functioning than content memory.
“We’ve got a group [of clients] who are not having content memory problems, yet they’re reporting, or their significant others are reporting, just as many difficulties in their everyday lives,” says Schmitter-Edgecombe. “These other aspects of memory may actually have greater contributions to what people are reporting as causing problems.”
In addition to temporal order memory, there’s source memory, which records where you got the information. When you tell a story to the person you first heard it from, that’s a failure of source memory. Your content memory is fine–you remember the story perfectly–but you’ve forgotten who told it to you.
Finally, there’s prospective memory, the ability to remember to do things in the future. Prospective memory involves long-term goals, like vacation plans, and daily details, like remembering to take medications at specific times.
It makes perfect sense, but I’ve never thought about memory like this before. Schmitter-Edgecombe says I’m not alone; there’s been very little research on non-content memory, especially in the context of dementia. That’s beginning to change. She and other researchers have found that serious problems often show up first with non-content processes. Temporal-order memory, especially, starts declining years before significant losses of content memory.
Schmitter-Edgecombe says that while the various forms of memory interact with each other, they largely reside in different regions of the brain. Content memory relies most heavily on the hippocampus, which lies fairly deep in the brain. Temporal ordering, source, and prospective memory involve higher circuits in the frontal lobes (behind your forehead), the parts of the brain responsible for decision-making or “executive ability.” She thinks that if we can understand how the different aspects of memory are related and how they affect people’s ability to get along in everyday life, we’ll have a better shot at developing strategies to compensate for memory deficits, and perhaps even delay the onset of memory-related problems.
She’s especially intrigued by “cognitive reserve,” the notion that staying mentally active gives us some degree of protection against dementia. People with lots of cognitive reserve still get Alzheimer’s, but they have excess capacity, a cushion, so they can still function well even with moderate deterioration in the brain. Eventually, the disease progresses so far that the reserve can’t help, and then the person declines with stunning speed. Still, delaying the onset of symptoms by a couple of years sounds good.
So, how can we tell if we have a sizeable reserve? Can we do anything to boost it?
To some extent, the die is already cast, she says. How much education you had, your language skills in early adulthood, and your genetic history all contribute. But there are some things we can do to improve it. Being socially active helps. So does aerobic exercise, keeping a healthy flow of oxygen to the brain. Mainly, we need to keep learning. More and more evidence suggests that we can pad our cognitive cushion through mental activity.
That’s what I was hoping to hear. My job requires plenty of mental heavy lifting, and at home I read challenging books, recently took up a musical instrument, and enjoy doing word and number puzzles. Is there merit in the suggestion I’ve read that working crosswords or Sudoku keeps the brain cells in shape?
Schmitter-Edgecombe thinks puzzles may help, as long as they require you to actively search for and integrate new information. Her colleague, biochemical psychologist Jay Wright, agrees, “but only if you’re really interested in doing it. Pick something that’s stimulating mentally, that you want to do. It could be reading, it could be solving things, it could be learning anything new that keeps you processing. You really need new experiences in order to keep things rolling.”
The human brain is made for problem-solving, says Wright. “We’ve got this cortex that requires us to use
it. I think that’s the key to aging. I think it’s anything that continues you problem-solving and trying to make sense out of something that challenges you.”
He says mental activity strengthens the brain’s ability to work around damaged areas and keeps brain cells alive longer, enhancing cognitive reserve. In Alzheimer’s disease, wads of protein gum up the neural connections and lots of brain cells simply die. We all lose brain cells as we age; people with Alzheimer’s lose more.
In particular, they lose cholinergic neurons, those that communicate via a neurotransmitter called acetylcholine. The drugs now used to treat early-stage Alzheimer’s all boost the amount or longevity of acetylcholine, which enables the neurons to keep talking to each other longer.
That’s OK as far as it goes, says Wright, but it ignores other chemical pathways that play a role in memory formation. Several years ago he and biochemist Joe Harding discovered one such pathway while researching how the body regulates its blood pressure. They were testing potential drugs that targeted molecules called angiotensin receptors and came across a receptor (AT4) that just wouldn’t cooperate. Turns out it’s absent from parts of the brain that control blood pressure but is abundant in parts that deal with memory.
Wright and Harding took a closer look at AT4 and found that it is, in fact, involved in memory formation. Their discovery helped explain a reported side-effect of certain blood-pressure medications. People who had taken the meds for several months had told their doctors, ‘oh, by the way, I feel a bit sharper mentally than I was before.’ That made sense, since maintaining a healthy blood pressure was known to be good for brain health. However, not all blood pressure medications had the same effect–only those that worked via the brain’s angiotensin receptors and activated AT4.
Wright and Harding ended up switching their research program from blood pressure to memory, which meant learning an entirely new literature and new experimental approaches. They found that the AT4 pathway works independently of the cholinergic system, and while its exact role isn’t yet known, they think it offers another possible target for drug therapy to reduce the symptoms of Alzheimer’s.
In one series of experiments, they are working to develop drugs that aid memory by “revving up” the AT4 pathway. To test their potential drugs, they have developed an animal model of dementia. (No naturally-occurring model exists.) They treat rats with a drug called scopalomine, which interferes with neurons that use acetylcholine. For the next few hours the treated rats behave in ways that look familiar to anyone who deals with dementia patients. In particular, they have trouble retaining new information. It’s not a permanent, progressive disease state, but a short-term, drug-induced inability to make new memories.
Wright and Harding test each rat by putting it into a round horse trough filled with water. There’s a small platform the rat can rest on, but it’s slightly submerged and not visible from the surface. Until the rat finds it, he has to keep swimming. Wright and Harding time how long it takes the rat to find the platform on successive days. Each rat gets one try a day, for eight days. “Demented” rats get scopalomine before each trial.
On the first day, not knowing the platform is there, the rats swim around for 90 to 100 seconds before finding it. Rats whose brains are functioning normally quickly learn where the platform is. Within a few days they swim more-or-less straight to it, reaching it in 15 to 20 seconds. A rat with drug-induced dementia, on the other hand, takes up to 75 seconds even after eight days of learning, and some never find it.
“That’s kind of what you see with people [who have dementia],” says Wright. “They can learn things, but they’ve got to rehearse it and you’ve got to go over it and over it with them, and even then the progress is really slow.”
In the key test of their potential drug, Wright and Harding gave the rats scopalomine to create dementia, and a new compound that enhances the AT4 system. After eight days of training, those rats found the platform in about 25 seconds–almost as fast as the untreated controls. It was a major finding: boosting AT4 largely compensated for the problems in the cholinergic pathway.
“Our hope is that maybe the drugs we’re developing would keep people alert and mentally active for another year, another two years, when they normally would be sliding,” says Wright. With one hand he sketches a sloping line in the air, indicating the decline in mental sharpness over time in dementia patients. “We’re not asking to stop the thing. I don’t think that’s possible. But to make the slope a little less [steep], so that they can still store new memories, retrieve those and old memories a little bit better.”
For some reason I’ve always thought of Alzheimer’s in terms of losing existing memories. Yet people with the disease generally retain their oldest memories the longest. Like Wright’s and Harding’s rats, it’s new memories they have the most trouble with, or integrating new ones with the old. And that means learning.
Wright says long-term memories are permanent in the sense that they last a long time, but they are not permanent in the sense of never changing. When you learn something new–create a new memory–you don’t simply add new information to old, like stacking books on a shelf. The brain actually remodels itself, its internal wiring, all the time. If new information contradicts an existing circuit, for instance, your brain must weaken those previous connections to accommodate the new stuff.
The mysterious part is that the remodeling happens right where you need it, right in the brain cells involved with that prior memory. If you’ve just learned a new route to the ballpark, you don’t want your brain changing the circuits that store the knowledge of how to cook spaghetti. How does your brain ‘know’ which connections to alter, which ones to strengthen, and which to do away with altogether?
Gary Wayman is working on those questions. He gets into the nitty-gritty biochemical details of how a signal from one nerve cell affects the growth and function of other nerve cells. “I’m a signal transductionist,” he says, using a term his mama surely never imagined for him. “I absolutely love dissecting these puzzles out.”
He also loves looking at nerve cells, or neurons, through the microscope. Healthy neurons are graceful things, with a roundish cell body that houses the nucleus, a long, sturdy axon that carries impulses toward other neurons, and an array of delicate branches called the dendritic arbor. Each dendrite, or branch, of the arbor has tiny hair-like spines that can meet up with an axon from another neuron to form a synapse. The synapse is the center of action in neural communication. A nerve impulse from one neuron travels down its axon to a synapse, where it crosses to a dendritic spine on another neuron. From there the impulse travels to the cell body, which determines whether to send the signal out through its own axon, or dampen the signal by not responding.
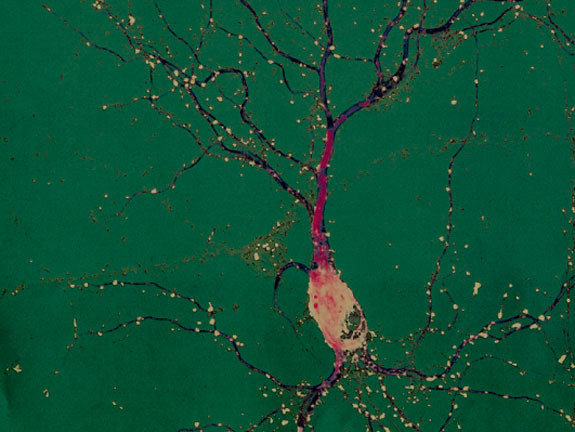
Wayman says the arbor is the key to much of brain health, including a robust memory. During infancy and childhood, as a child explores her environment, a flood of new information stimulates her neurons and the dendritic arbors grow. Dendrites that are used a lot branch more; dendrites that don’t carry many signals get pruned back, as the neuron focuses its effort on the connections that are used most often.
Wayman starts with an experimental setup that shows clear links between how active neurons are and how connected and healthy they are. He puts neurons from a young rat brain into a culture dish and lets them grow a while to establish connections with each other. Then he adds the drug bicuculline, which prompts the neurons to transmit s
ignals to each other as they would normally do in response to touch, sight, or whatever else the rat was experiencing.
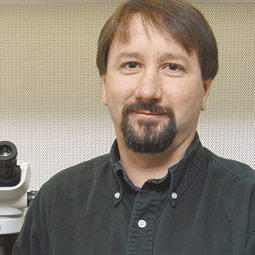
The arbors of neurons that receive signals from other neurons grow. Some dendrites get longer; others branch more. They also establish more synapses with neighboring neurons. It’s exactly what you see in a young brain as the animal it belongs to learns about the world around it. That much has been known for years, but the molecular mechanism for it has been a mystery.
“They knew that if you increase synaptic activity, you modulate the dendritic arbor, you affect the formation of synapses,” says Wayman, “but nobody knows what’s going on inside the cell. That’s where we came in.”
He and his colleagues at the Oregon Health and Science University have traced much of what happens inside a neuron as a result of incoming signals. A welter of chemical details boils down to this: Dendrites contain a protein that inhibits their further growth. Synaptic activity blocks the protein and moves it away from the active site. Synaptic activity also prompts the neuron to make a tiny RNA molecule called micro RNA 132 (miR132), which suppresses the production of more inhibitor. When miR132 production goes up, there’s less inhibitor and the dendrites grow more in areas with active synapses. When Wayman blocks miR132, more inhibitor protein is made and dendrite growth stops or slows down (see an illustration link below).
My head is spinning. Boosting growth by inhibiting an inhibitor seems overly complicated, I say.
“Is it complicated?” asks Wayman. “Or is it giving the cell a means of fine tuning?” He reminds me that the conventional view of how cells work holds that a signal turns on genes in the nucleus, which leads to the production of messenger RNA and, eventually, of protein. That works for many cellular functions, but for the delicate job of making highly specific connections with other cells, it won’t do. If a neuron’s nucleus pumped out RNAs that directly promoted growth, all of its dendrites would grow, not just the ones at the site where the synaptic activity occurred.
And that’s the key part of the system. When we learn something new, we don’t get a wholesale activation of neurons throughout the brain. Or we shouldn’t, at any rate. The last thing we want is general sprouting throughout the brain, says Wayman; that way lies madness, almost literally. Indiscriminate nerve firing causes seizures and has been linked to some forms of retardation and to loss of memory.
By having the molecular machinery for dendrite growth already in the dendrites, but in an idle form, a signal at that site can activate the machinery in that locale alone, not throughout the neuron or brain as a whole.
The final piece clicks into place. It fits what Wright told me about remodeling, it fits what he and Schmitter-Edgecombe said about giving our brain cells new problems to chew on, it fits what’s known about more active brains suffering less neuron loss. Doing a hard mental task stimulates neural activity, which encourages neuron growth and connection. As Wayman says, “an active nerve cell is a cell that’s going to live another day.”
So I’m all set with an action plan for brain health. After work today I’ll practice a new song, call my best friend, and go for a brisk walk with the dogs.
Oh, and where did I leave that new Sudoku book?
Web extra
DISCOVERY :: Testing 1 2 3, online tests for different aspects of memory
Maureen Schmitter-Edgecombe’s work to help people with memory loss
Illustrations
Jay Wright and Joe Harding’s experiments on chemical pathways that play a role in memory formation.
Gary Wayman’s research on how activity stimulates localized dendrite growth in neurons.
On the web
Video: Maureen Schmitter-Edgecombe on Specific MemoryvImpairments in Dementia and MCI (GoCognitive.net/YouTube)
Video: Maureen Schmitter-Edgecombe on Alzheimers, Dementia, and Mild Cognitive Impairment (GoCognitive.net/YouTube)
Video: Maureen Schmitter-Edgecombe on Intervention Strategies in MCI and Dementia Patients (GoCognitive.net/YouTube)