AS WOMEN NEAR 40 YEARS OF AGE, their chance of having a miscarriage or a child with a serious disability or birth defect increases more than ten-fold. Most of those problems are due to defects in the baby’s chromosomes, the strands of DNA that carry a person’s genetic inheritance. Down syndrome, for instance, results from trisomy 21—three copies of chromosome 21 (there should be just two).
Most such problems arise in the egg, and the older a woman is when she conceives, the more likely it is her eggs will have abnormal chromosomes.
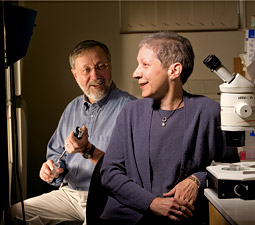
But amid all the talk about the biological clock, we often overlook a bigger story: that even with young mothers, chromosome abnormalities are the single most frequent cause of miscarriage and birth defects.
“Somewhere around 25 to 30 percent of all fertilized human eggs don’t have the right number of chromosomes,” says Terry Hassold, a human geneticist at Washington State University. “A huge number is selected [out] in very early pregnancy. It comes across as a missed period or a late period. But it’s actually a conception.”
The high error rate seems to be peculiar to humans. In other mammals that have been studied, the figure is at least 10 times lower. Hassold shakes his head in wonder.
“As many times as we’ve talked about this, it really is kind of astonishing.”
HASSOLD, BEARDED AND GENIAL, is one of three researchers at WSU who have devoted their careers to tracking down the sources and effects of chromosome abnormalities. Pat Hunt, his research partner, is a developmental biologist with a wry sense of humor and an elfin gleam in her eye. They married while training in one of the world’s top chromosome labs, and have been studying chromosomes ever since. Lisa Shaffer (’84 Biology), a human geneticist, came to WSU Spokane in 2002, after working in Texas for several years, because the Northwest is home. She grew up in Kennewick.
Many medical researchers choose their field because it relates to events in their own life. That’s not the case with these three.
Hunt was driven by a fascination with “the nuts and bolts” of how eggs develop and what makes the process go wrong, especially in women approaching menopause. For Hassold, the initial draw was aesthetic. His doctoral research at Michigan State included an examination of chromosomes.
“And I fell in love with them,” he says. “Just seeing human chromosomes, and—this is going to sound really saccharine, but—just being amazed by how pretty they were.”
Shaffer caught the chromosome bug when she was a sophomore in high school. One day her biology textbook opened to a picture of a child with Down syndrome. “I decided right then and there, that’s what I was going to work on,” she says. “I had never even met anyone with Down syndrome. I saw that and it fascinated me that having more of our genetic materials—having an extra chromosome—actually causes problems. You know, everyone’s always telling you ‘more is better.’ But when it comes to genetics, more is not necessarily better.”
GETTING IT RIGHT
Having the wrong number of chromosomes, a condition called aneuploidy, is almost always fatal to an embryo. (Even trisomy 21; about 80 percent of embryos with that abnormality miscarry. “So those infants that are born with Down syndrome represent a rare group of survivors,” says Hassold.) Our systems are set up to run with two copies of each chromosome, one from each parent. Think of it as the Goldilocks principle—you don’t want too little genetic material and you don’t want too much. “You want it just right,” says Shaffer.
Getting it just right for a new embryo requires perfect execution of precise steps in the developing eggs and sperm. Each has to reduce its chromosome complement by half, retaining one member from each of the 23 pairs of chromosomes. That way, when egg and sperm get together at fertilization, the resulting embryo will have a perfect set.
As Hassold describes it, the parceling out of chromosomes in developing eggs and sperm looks like a dance involving dozens of couples. The chromosomes line up in the middle of the cell, each one alongside its partner. The partner chromosomes “cross over”?—their arms literally cross each other—and swap segments of DNA. Eventually, when the cell divides, the partners move in opposite directions, so that each daughter cell will get one member of every chromosome pair.
This is where things are most likely to go wrong. If the partners stick together too tightly, they may not be able to separate from one another, or they may break when they try to pull apart. If they stick too loosely or never find each other in the first place, they will behave as individuals rather than as a matched pair. About half the time, they’ll go the same direction during cell division rather than splitting up. Such errors can produce an egg or a sperm with a damaged chromosome, an extra copy of a chromosome, or no copies at all.
Prospective sperm and eggs (spermatocytes and oocytes) go through most of the same steps, with one big difference that might explain why eggs are much more likely to have chromosome abnormalities. Sperm development starts at puberty, and once spermatocytes start the process, they go right on through to being functional sperm. Eggs start to develop when the future mother is an embryo. About 10 weeks into gestation, oocytes inside a female human fetus begin the long process of becoming eggs. Their chromosomes pair up and cross over. Then the oocytes arrest. They simply stop developing. Their chromosomes hold their positions, sometimes for decades, until hormones tell them to finish the dance and complete cell division. Every egg ovulated by a grown woman has been sitting in a state of suspended animation since the woman was an embryo about the size of an adult’s thumb.
That delay may be the source of many chromosome problems in older mothers. Hunt and Hassold have found that a protein that holds the partnered chromosomes together might play a role in age-related errors. Mutant mice that make a defective form of the protein have a high rate of aneuploidy. In women, the protein might get damaged during the long period of arrest.
While they were doing the painstaking experiments to find what makes good eggs go bad in older mothers, something wholly unexpected turned up. Hunt’s lab saw a sudden increase in chromosome abnormalities in eggs from young adult mice that were supposed to be the normal, control animals in a study.
“We ran the experiment one week and the control data were normal,” recalls Hunt. “The next week, data from the same control animals were completely different and unlike anything we had seen before.”
After ruling out every other factor she could think of, Hunt traced the problem to the cages the mice were housed in and the water bottles they drank from. Both were made of polycarbonate plastic, which is a polymer (long chain) of a small synthetic chemical called bisphenol A (BPA). The week before the mice started making defective eggs, the cages and bottles had been washed with the wrong cleaning agent. It damaged the polycarbonate, allowing BPA to leach out.
The discovery launched Hunt on a crash course in BPA toxicology. BPA already had a reputation as a health hazard. It’s a mimic of the female hormone estrogen, which allows it to affect many systems in the body. As a component of polycarbonate, it’s all around us: in plastic food containers, sippy cups, toys, shampoo bottles, resins that line aluminum food cans, you name it. Over the past decade, low levels of BPA—levels many of us already carry in our bloodstreams—had been linked to low sperm production, altered brain structure and behavior, and some forms of cancer.
Hunt added to that list an effect on chromosomes—and on future generations. She exposed mice to BPA at different times of life and different stages of gestation. It caused problems in adults and in newborns. Most startling of all was its effect on embryos
in which the eggs were just starting to develop. Forty percent of eggs that were exposed to BPA at that stage ended up with either too many chromosomes or too few.
“It’s amazing,” says Hunt. “We can disrupt everything with this little pulse of BPA in this critical window of development. You hit the mom, you’re hitting her [embryonic] daughter or son; and if it’s a daughter, you’re hitting her developing eggs, which will be the [mom’s] grandchildren.
“So by exposing the mom, you’re affecting the likelihood that the grandchildren are going to be chromosomally abnormal.”
It’s likely that BPA affects humans the same way, says Hunt. That means that a girl fetus exposed to BPA might have normal chromosomes in most of her cells, but when she grows up and tries to have children of her own, they will have a high risk of being chromosomally abnormal, because the eggs they developed from were damaged by BPA way back when their mother was a fetus.
Hunt’s work on the effects of BPA on developing eggs was the last straw in a pile of evidence that finally broke through into public awareness. Her findings made headlines all over the world, and Scientific American named her one of the top 50 researchers in the world in 2007. With awareness came action: Canada has banned the use of BPA in baby bottles, several U.S. states are considering similar measures, and a congressional committee has started an investigation of BPA. Baby-bottle manufacturers aren’t waiting for new regulations; they’re already shifting production toward materials that don’t contain BPA.
The attention on her has been a bit much, says Hunt, who cites the contributions by the dozens of other scientists working on the dangers posed by BPA. What makes her new prominence palatable is her conviction that BPA is, in fact, very scary stuff.
“When you see the profound effect it has on the mouse, you think, wow,” she says. “It gives me pause.”
BPA has actually turned out to be a handy tool—a monkey wrench, Hunt calls it—that lets her tinker with early fetal events and get a better look at how chromosome abnormalities occur. Mice have never been a great model system for this problem, she says. “As young reproducing females, they have a really low level [of aneuploidy] compared to us. It’s like one or two percent. With age it doubles. Big whoop.” By increasing the incidence of aneuploidy, BPA makes mice a better model system for studying how the chromosomal choreography might go wrong in humans. She and Hassold have already found that BPA boosts the number of crossovers between partners, supporting their hunch that having too many crossovers makes the chromosomes more likely to stick together when they should be separating.
“It’s so cool,” says Hunt. “How would we ever figure that out [without BPA]? We wouldn’t.”
COMPLETING THE PICTURE
After working for several years on the causes of chromosome abnormalities, Lisa Shaffer now focuses on their consequences. She’s especially interested in subtle abnormalities such as deletions, in which a small piece of a chromosome is snipped out and lost. Like aneuploidy, most deletions can be traced to mistakes during early egg development. Unlike aneuploidy, they seem to occur as often in young parents as in older ones.
They’re also harder to pinpoint. Aneuploidy is fairly easy to diagnose: drench the cells in a chromosome-specific stain, put the stained cells under a microscope, and count the chromosomes. That technique can reveal huge deletions, but it rarely catches those smaller than 10 megabases, a length of DNA that could encompass dozens of genes.
Shaffer detects losses a fraction of that size with a technique called microarray analysis. A microarray is the size of a standard microscope slide. Spotted on it are known segments of human DNA. To run a test, the lab crew puts on the slide small samples of the patient’s DNA and DNA from a known, control individual. Each sample carries a fluorescent label: green for patient DNA, orange for control DNA. The two samples compete to bind with their matching sequences in the spots on the slide. If the samples have the same number of copies of a segment, the matching spot will fluoresce yellow (combination of the green and the orange.) If the patient has a deletion—one copy of a segment instead of two—more of the control DNA will bind and the spot fluoresces orange. If the patient has extra copies of a segment, the matching spot fluoresces green. A tidy graph of the color of each spot shows clearly where the patient and control DNA differ.
In 2003, Shaffer and fellow WSU Spokane geneticist Bassem Bejjani put microarrays to work diagnosing chromosome and genetic abnormalities for their new company, Signature Genomic Laboratories (see sidebar, “Trust your crazy ideas”). They do some pre-natal testing, but most of their clients are doctors who are treating a child with mental retardation or birth defects for which the source of the problems has not been identified. It might seem too late to run chromosome tests on a 6-year-old, but Shaffer says that’s not the case at all.
“It’s important to make a diagnosis,” she says. “The first reason is because parents want to know what’s wrong with their child. They also need to understand how it happened, because they may be at risk for it happening again, or their family members may be at risk for having a similar child. And then for some chromosome abnormalities, there are certain medical problems you need to anticipate. By anticipating them, the child can receive treatments and have a better quality of life.”
A few years ago, she discovered that children with a deletion called 1p36 (loss of DNA from band 36 on the short arm [p] of chromosome 1) had poor hearing. That was a major finding, because many kids with a 1p36 deletion don’t talk. “Maybe they don’t speak because they can’t hear,” says Shaffer. “If they have hearing problems, let’s get them hearing aids so they can hear, and hopefully they’ll develop speech.”
She is now exploring 1q42 deletions (loss of DNA from band 42 on the long arm [q] of chromosome 1). The company’s tests have identified nine children with this deletion. With the permission of their parents, Shaffer took a closer look at the symptoms and genetic characteristics of seven of them and one other child from a previous study.
She found that although their deletions are in the same region of the chromosome, the kids aren’t all missing exactly the same chunk of DNA. They also don’t have exactly the same symptoms. All have mental retardation or developmental delay, but it ranges from mild to severe. All have defects in structures along the body’s midline, such as cleft palate; but again, some have much more severe problems than others.
By diagramming the eight deletions and lining them up next to a map of the intact chromosome, Shaffer was able to identify what she calls their “smallest region of overlap” (SRO)—the piece all of them are missing. That piece accounts for the symptoms the children have in common. It contains only five genes, all of which have been identified. Shaffer also found that the child with the mildest symptoms is missing a big piece to the left of the SRO but little to the right, while the child with the most severe symptoms is missing a big piece to the right of the SRO but little to the left. That’s a clue that at least one gene to the right of the SRO is involved in the more severe symptoms.
Shaffer is eager to find out more about the genes in and around the SRO. “That’s really exciting, to bring all the pieces together,” she says. “The genes are the final piece. We have the deletion, we’re dealing with that—but what’s missing, and why is it important? If we can identify the gene targets, maybe there’s a treatment.”
RISKY BUSINESS
Being able to alleviate the effects of a chromosome abnormality would be a huge benefit to the children and families involved, but the ultimate hope of chromosome research is that by understanding
how abnormalities happen, we can find a way to prevent them or at least make them much less common. What can prospective parents do to reduce the chance that their child will have a chromosome abnormality?
Unfortunately, we don’t know yet, says Hassold. Stacks of studies have been done looking for risk factors; none have resulted in solid guidelines.
That’s not to say there aren’t risk factors. It’s just that with most chromosome abnormalities arising when the mother was a fetus, how do researchers even begin to trace what caused them?
Hunt’s work with BPA offers the one firm piece of advice that holds for women—and men—of any age who want to lower their risk of one day having a grandchild with a chromosome abnormality: rid your life of as many BPA-containing products as you can, especially in the kitchen. Use glass or ceramic containers instead. If you do use plastic or polycarbonate containers, don’t expose them to high heat. That means they never go in the dishwasher or microwave.
Beyond that, there’s no prescription. Hunt, Hassold, and Shaffer all are convinced there is no single cause of chromosome problems, and no single measure that could prevent them. Chromosome abnormalities can’t be chalked up to anything either parent did or didn’t do—which can be comforting news to parents of a child who has a chromosome problem.
“I think it helps for families to understand that this wasn’t [due to] anything that they did, and it wasn’t something that could have been prevented,” says Shaffer. “It’s just something that happened, and we don’t understand why, and it’s a rare event.”
Terry Hassold sums it up.
“Sadly, the risk factor is being a human female. That’s the risk factor.”